By: MSc(c) Gastón Morales, Research and Development Engineer at Digital Sense; in collaboration with Prof. Gabriele Facciolo at ENS Paris-Saclay; MSc Luis Di Martino, Team Leader at Digital Sense; PhD Javier Preciozzi, Managing Partner at Digital Sense.
A problem that may become increasingly common
Since October 2022, Uruguay has been experiencing a historical water deficit, leading the government to declare an agricultural emergency [1], which has been extended until now (May 2023) to alleviate the consequences of the lack of rainfall on production.
In January of this year, the situation became almost untenable for the State Sanitation Works (OSE), the state-owned company with a monopoly on water in the country. The company had to issue the first official alert, urging "responsible use" of water [2].
In February and March, the situation did not improve. OSE called on the population again to "consume drinking water responsibly" as there was a high risk of water shortages in various locations. The drought continued unabated, and the Canelón Grande reservoir ran dry [3]. OSE lost one of its four reserves for supplying Montevideo and its surroundings.
In April, there were rains that alleviated the situation, but not enough to lift the agricultural emergency. According to information from the Uruguayan Institute of Meteorology (Inumet), Uruguay remains under drought conditions. In fact, the extreme southwest and south of the country are "under conditions of extreme drought with less than 15% of available water in the soil" [4].
By the end of April, the situation in the metropolitan area reached a new critical point due to the lack of rain and the depletion of reserves. OSE started treating water with higher salinity levels, mixing water from the Paso Severino reservoir, which is freshwater, and the lower stretch of the Santa Lucía river, which has higher salinity levels due to its proximity to the mouth of the Río de la Plata [5]. To do this, OSE had to request a temporary exception from the Ministry of Public Health to increase the maximum allowable salinity values for the metropolitan area.
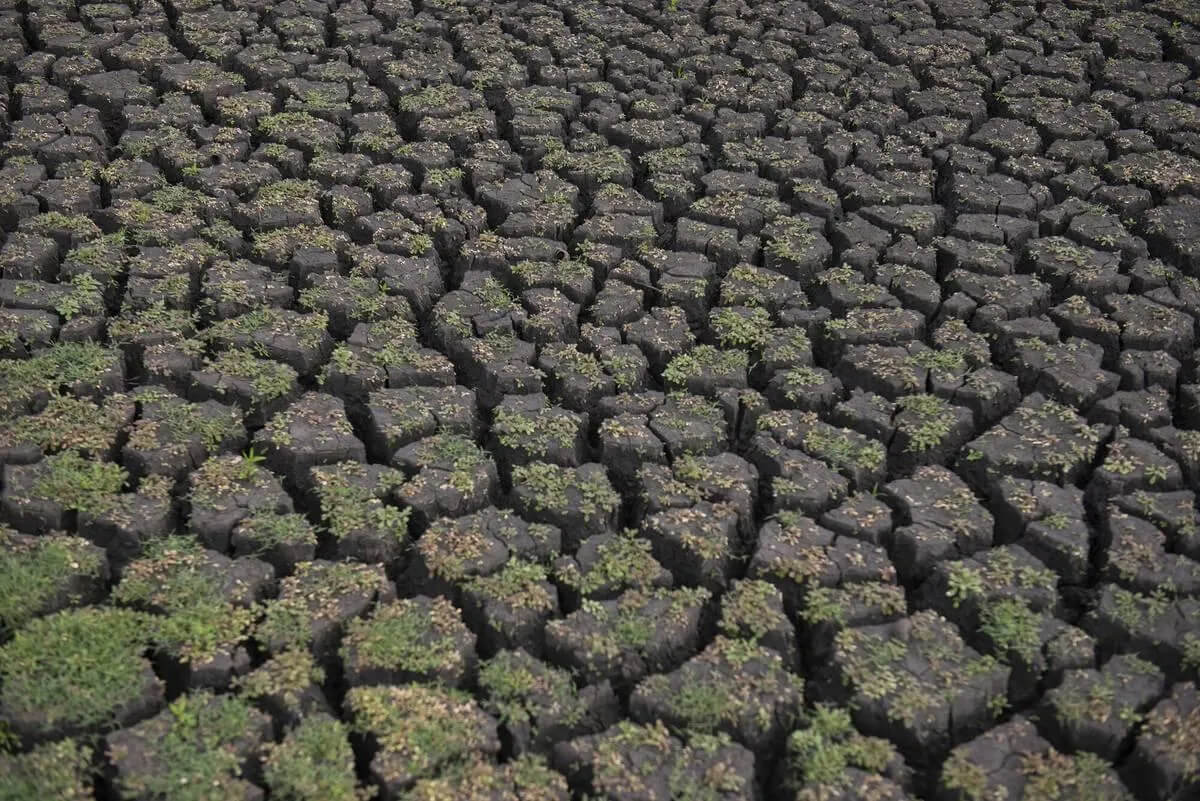
The country and its resources
Uruguay is known for its significant access to potable water, an increasingly precious resource globally [6]. With substantial natural access points and water treatment plants, the country usually does not face major issues regarding drinking water. However, the droughts of the summer of 2023 have raised alarms for OSE, as historic lows were reached in the reservoirs supplying Uruguay's main metropolitan areas.
OSE operates eight types of water treatment plants [7], depending on the primary water source: the basin of the Negro river, the basin of the Río de la Plata, the basin of the Santa Lucía river, the basin of the Uruguay river, the basin of Laguna Merín, the Atlantic Ocean, sources from underground, and osmosis treatment plants. The two most significant reservoirs for the metropolitan area are Canelón Grande and Paso Severino.
How can technology help in this problem?
Water availability is a critical issue that can severely impact a country; therefore, estimating how much water is available at all times is of utmost importance.
At Digital Sense, we aim to leverage the capabilities of Image Processing, Remote Sensing, and Machine Learning to apply them to water monitoring in this case.
In this study, we use Synthetic Aperture Radar (SAR) satellite images to monitor Uruguay's water bodies under any climatic conditions precisely.
Furthermore, we estimate the water quantity in a reservoir based on image processing and data analysis, combining them with bathymetric data supplied by OSE.
Why SAR?
SAR technology is increasingly used for terrestrial monitoring due to its numerous advantages compared to other Earth Observation technologies.
A SAR satellite emits a radar signal toward the Earth's surface and then receives the reflected signal by measuring its return time. This signal is processed to generate an image of the Earth's surface based on its amplitude and phase, as shown in Figure 1, from which information about topography, vegetation, water presence, and other surface elements can be extracted.
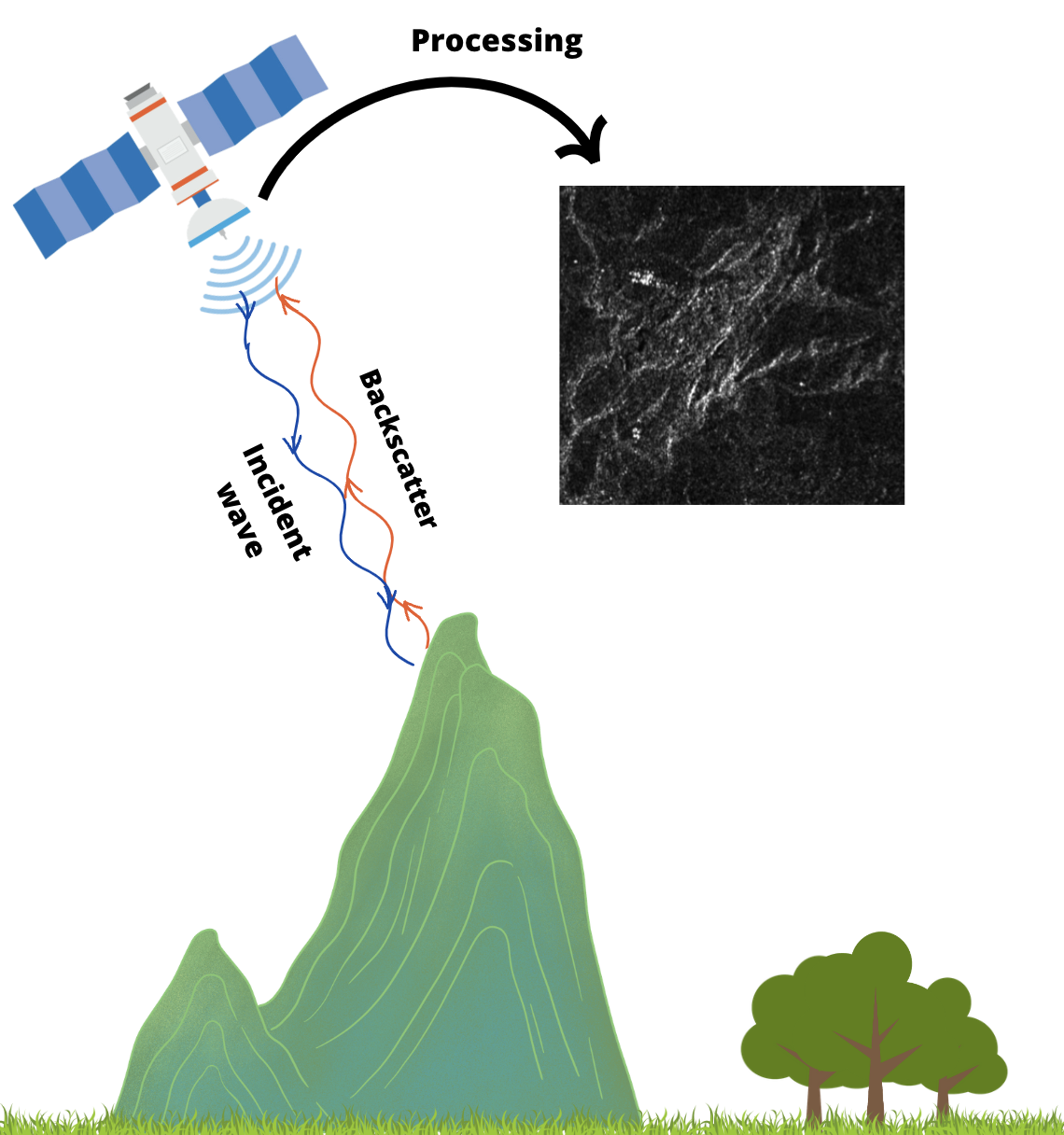
SAR technology has the advantage of being able to observe in any weather condition. Unlike optical satellite images, which the presence of clouds or fog can hinder, SAR images have the ability to penetrate through these conditions and provide clear and detailed images of the Earth's surface. Figure 2 shows the Paso Severino reservoir with optical and SAR images on a cloudy day.
Moreover, satellite images can be taken during the day and at night, allowing for continuous monitoring.
Depending on the instrument, SAR images can provide relatively high spatial resolution, between 1 and 10 meters per pixel. This allows for detecting small objects and detailed Earth surface analysis.

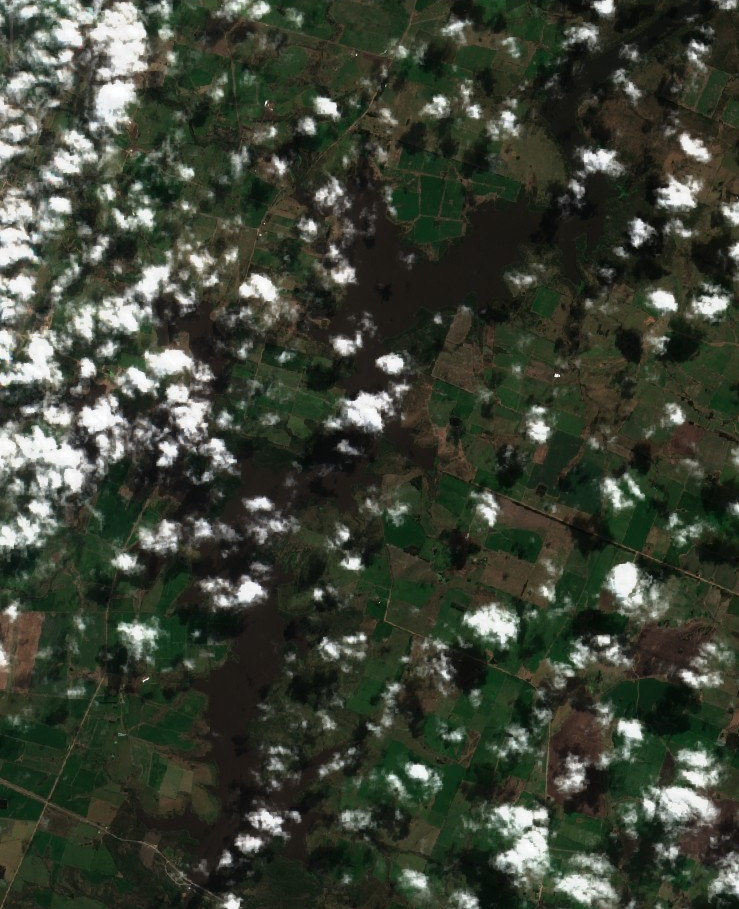
The data
The study focused on changes in water levels in two of the main water supply sources for OSE.
Paso Severino Reservoir: located in the lower course of the Santa Lucía Chico River in the department of Florida (34°15′56.74″ S, 56°18′24.54″ W).
Canelón Grande Reservoir: Uruguayan river that crosses the Canelones department (34°30′31″ S, 56°23′27″ W).
To complement the study, a third reservoir used for irrigation was added:
India Muerta Reservoir: located between the towns of Lascano and Velázquez, in the department of Rocha (33°55′16″ S, 54°15′22″).
The satellite used for this study was Sentinel-1, belonging to the Copernicus program developed by the European Space Agency (ESA) [8]. This satellite provides high-quality SAR image data with global coverage.
There are several synthetic aperture radar satellites in addition to Sentinel-1, such as TerraSAR-X or COSMO-SkyMed. The advantage of the former is that its data is free and easily accessible. In our case, the images were extracted through Google Earth Engine, a Google platform for processing and analyzing geospatial data.
Sentinel-1 SAR satellite images have a spatial resolution of up to 10 meters. Their ability to penetrate through the atmosphere, clouds, and rain allows for continuous observation of the Earth's surface, even in adverse weather conditions. This provides a revisit time of 12 days for most land areas.
Specifically, the images used for this work belong to those acquired in its descending orbit, with the Interferometric Wide (IW) mode and with transmission and reception of the wave with vertical polarization (VV).
Our work
Using SAR images obtained from the amplitude of the received signal, an analysis was conducted on how water levels varied in different reservoirs.
The signal reflected by calm waters, such as reservoirs, is considerably lower than that reflected by the rest of the surface, as water acts as a "mirror," reflecting the wave in a direction different from that of the satellite. This makes it appear darker in the SAR image. On the other hand, rough surfaces appear brighter since they reflect the radar wave in all directions, with a larger portion of the reflected wave returning to the satellite. Figure 3 shows a simple representation of this effect.

Based on this, water segmentation is performed using a threshold that separates water from the rest.
Normalization is carried out to compare a collection of SAR images of the reservoir and perform segmentation with a single threshold for all, bringing pixel values from different images into comparable ranges. This process aims to make all images in a collection have a similar appearance, eliminating differences due to variability in SAR image acquisition.
The normalization process follows these steps:
- Select an invariant reference area, an image region that does not change significantly between acquisitions. Calculate the mean pixel value in this reference area for a reference image.
- For each of the remaining images in the collection, calculate the mean pixel value in the invariant area and compare it with the mean pixel value of the reference image.
- Use the difference between these values to adjust the image by adding or subtracting this difference to all pixel values.
- Finally, apply filtering to remove image noise, making segmentation more precise.
Figure 4 shows water segmentation with the same threshold for two different dates.
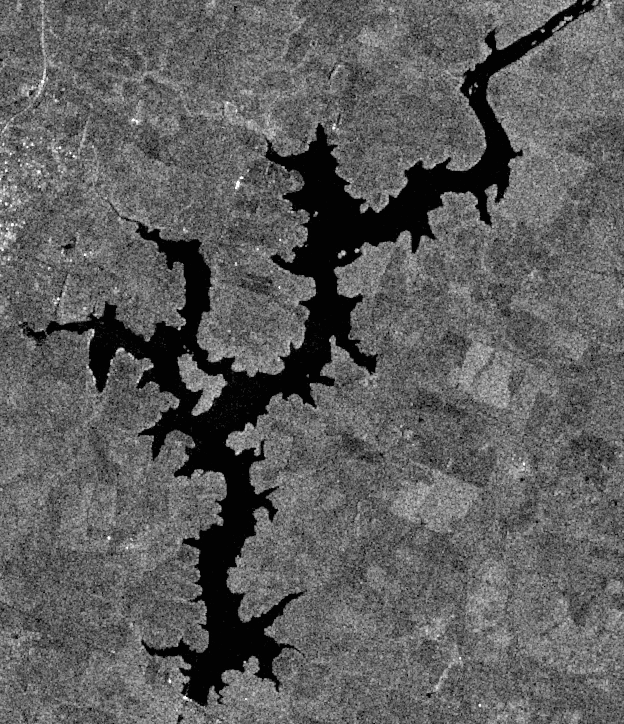
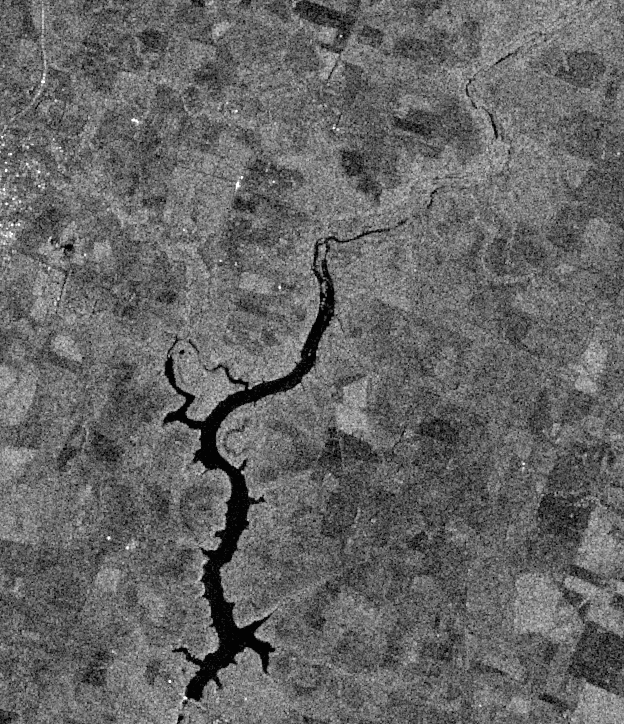
After processing the SAR image collection of the reservoirs, the drought's impact over time becomes apparent.
With segmentation data, the number of water pixels in each image is obtained, which can be directly mapped to square meters using the image's resolution.
Figure 5 illustrates how reservoir levels vary over time with their respective estimated water mirror values. Note that the described method only allows estimating the water surface area, not the available water volume. To determine water volume, additional information such as the bathymetry of each reservoir is needed. However, if the water surface area decreases, the volume does as well.

In all three cases, the effect of the drought is observed from January 2023 onwards, as the amount of water in the reservoirs significantly decreases.
Combining the study with bathymetry data provided by OSE for the Paso Severino reservoir allows estimating the available cubic meters of water.
The water situation on 03/22/2023, as reported by OSE, indicated Paso Severino had a reserve of 18,849,167 cubic meters of water [9]. Based on water detection from an image on 03/23/2023 and using bathymetry data, an estimate of 18,149,582 cubic meters is obtained.
Figure 6 shows water detection on 03/23/2023 and overlays it with bathymetry data.
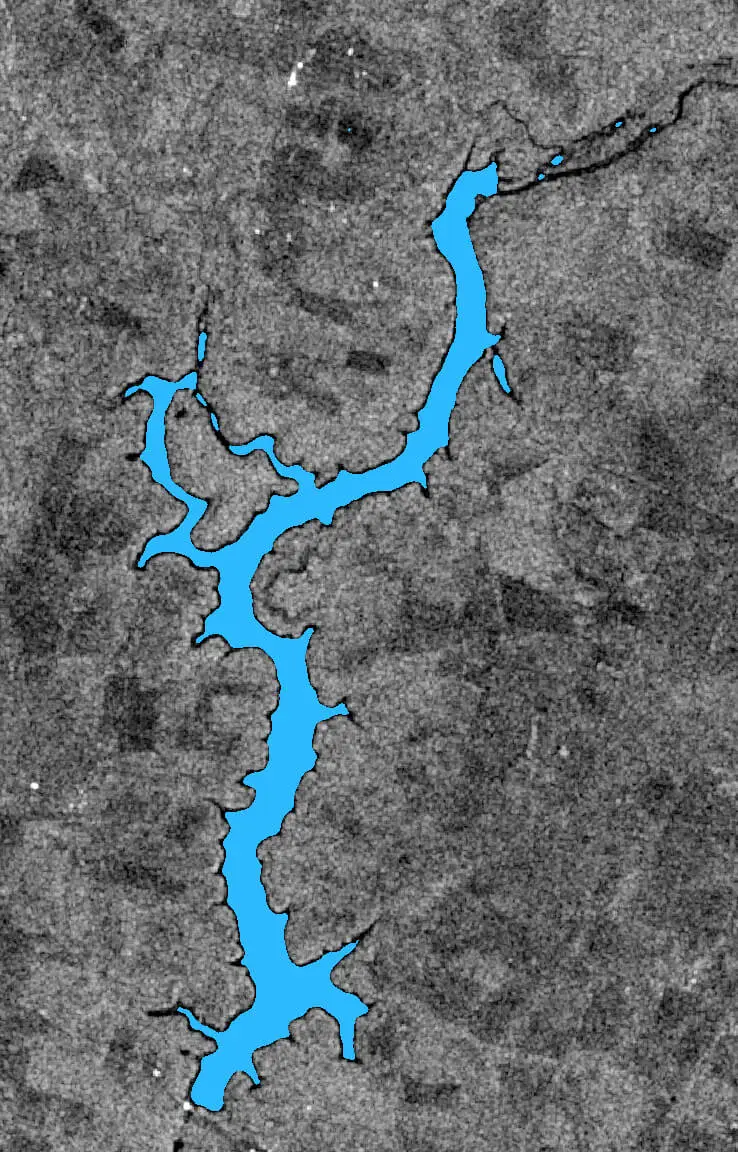

Furthermore, analyzing the evolution of the water mirror of Paso Severino in the last five years reveals two marked events: the current drought and that of 2020. Figure 7 clearly highlights the severity of the current drought, reaching a minimum far below that of 2020. Figure 8 shows images of the corresponding minimum points on 06/12/2020 and 05/10/2023.
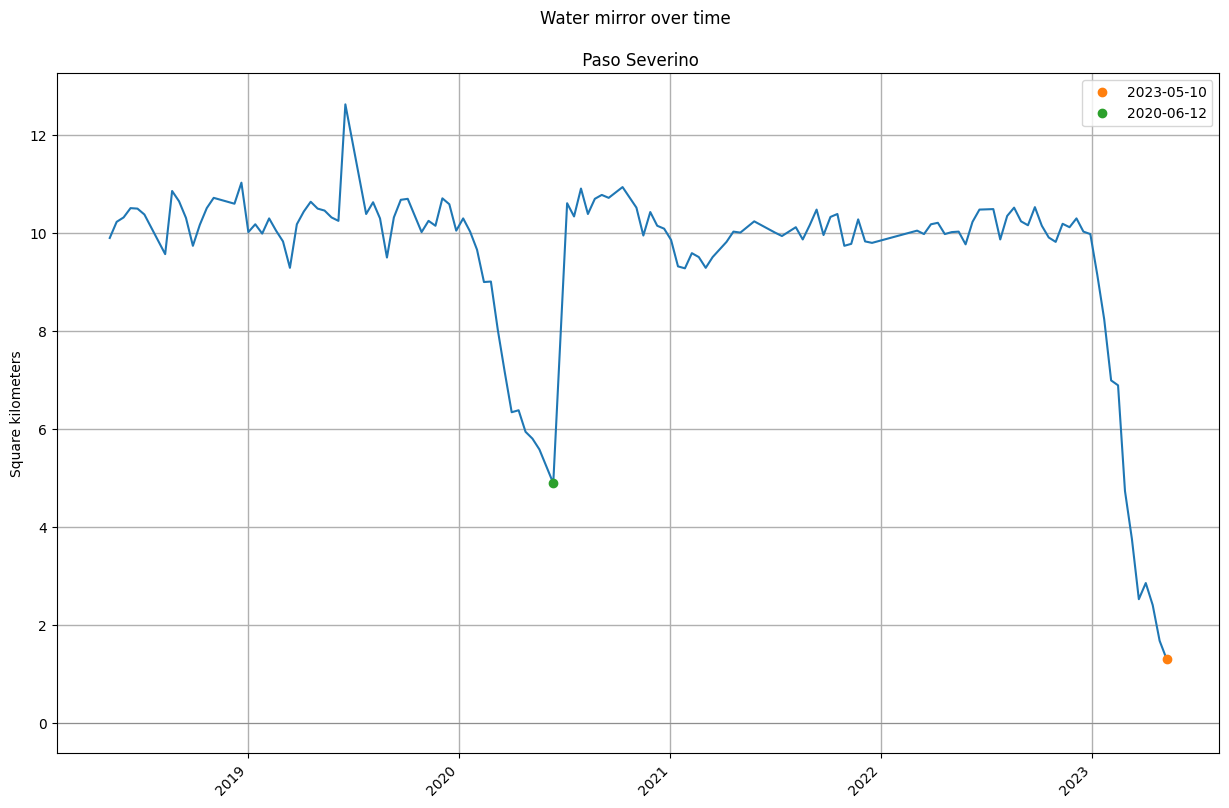

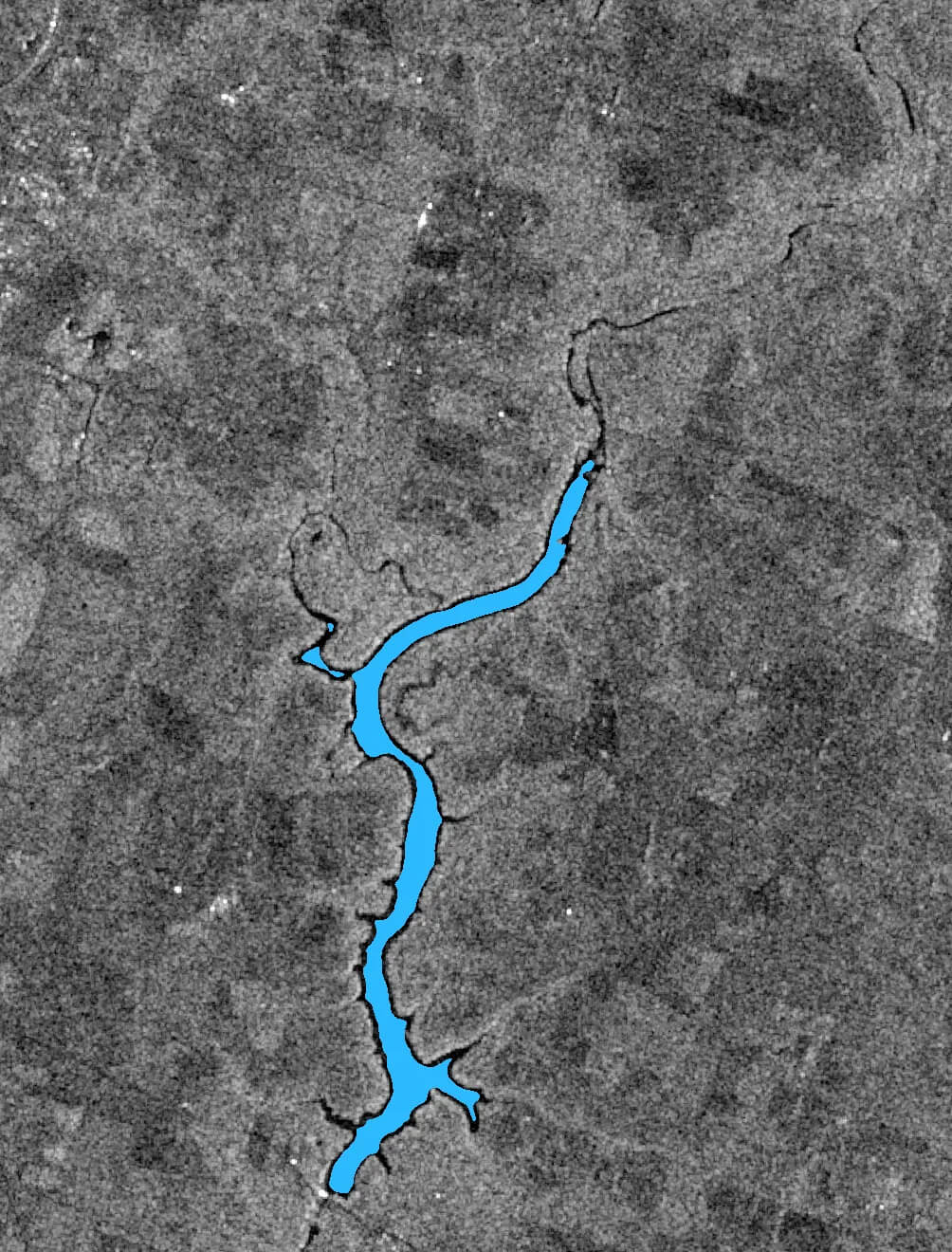
Conclusions
The problem of water scarcity may become increasingly common, emphasizing the need for continuous monitoring. The water and agricultural emergencies faced by Uruguay have highlighted the necessity for new technological solutions to foresee such situations. Through satellite detection algorithms, an automatic estimation of the available water quantity could be achieved.
It's essential to note that this work focuses on three specific reservoirs but can be extended to cover the entire country.
This work provides a proof of concept of how SAR satellite imaging technology allows for water monitoring. Our experiments illustrate the severity of the 2023 drought and compare it to the drought of 2020.
The Digital Sense team continues to work on research and development in this field, exploring various possibilities to contribute to addressing the concerns of the country.
References
[1] Agricultura y Pesca. Estimaciones preliminares impacto déficit hídrico 2022–2023; Ministerio de Ganadería.
[2] Debido a la sequía, OSE exhorta al “uso responsable” del agua; La Diaria.
[3] Por la sequía, el embalse de Canelón Grande se quedó sin agua y OSE pierde una reserva: las impactantes imágenes; El Observador.
[4] Continúa la sequía en Uruguay; Inumet.
[5] Salinidad del agua en Montevideo y área metropolitana; Unidad Reguladora Servicios Energía Agua.
[6] El valor del agua; UNESCO.
[7] Ubicación de Plantas Potabilizadoras en Uruguay; OSE.
[8] Sentinel-1 SAR User Guide; European Space Agency.
[9] Déficit hídrico | Estado de situación al 22/03/2023; OSE.